|
abstract
In our laboratories, we study the electron-impact excitation of atoms and molecules.
We do this by observing the light emitted by the atoms (or molecules) as they decay
from the states into which they were excited into by the electrons. The results
we generate are called cross sections. We determine the cross section for electron-impact
excitation into given atomic/molecular levels as a function of the incident electron's energy.
Electron Impact Excitation
In our work, we start with a collection of target atoms (or molecules). In most
cases this is simply a vacuum chamber that has been back filled with the gas
of interest. However, we also do experiments on metastable atoms-- where the
target atoms must first be prepared in an excited state. A mono-energetic
electron beam is passed through the taget atoms. Some of the target atoms are
excited into higher lying levels. These excited levels eventually decay giving
off light whose wavelength depends upon the exact upper state that was
populated. By measuring the light from the target atoms at each wavelength
we find the cross sections for excitation into each of the upper levels.
Measurement of Optical Emission Cross Sections
One must keep in mind that there are two ways to populate an excited state:
1) | Direct excitation from the ground state |
2) | Cascade from excitation into higher lying levels |
By using the method described above for measuring optical emission cross sections,
we are actually measuring the sum of both the direct excitation cross section out
of the ground state plus the contribution due to higher lying levels cascading
into the level of interest.
Since the direct excitation cross section is usually desired, one must determine the
total cascade contribution and subtract this from the apparent cross section.
Excitation Functions
An excitation function is a plot of cross section versus the incident electron
energy. Excitation functions for the direct cross section generally fall into a number of catergories,
depending upon what the final (excited) state is. For example, in electron impact
excitation of ground state helium there are three families of excitation functions:
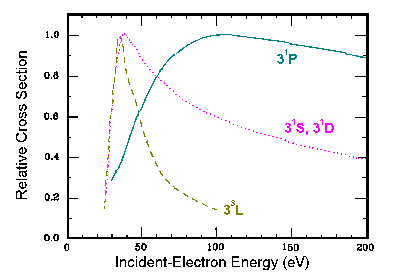
1) |
11S to n1P |
Broad excitation function, with peak around 100 eV. |
2) |
11S to n1S,n1D |
Narrower excitation function, with peak close to onset. |
3) |
11S to n3L |
Sharply peaked, with rapid ~1/E3 fall off in tail. |
This general energy dependence can be understood in terms of selection rules.
An electron at very high velocities/energies generates an electric field similar
to a photon. Thus, excitation into the optically allowed n1P levels is
larger at high energies than excitation into the dipole forbidden n1S
and n1D levels. Excitation into the n3L is controlled by
the target atom capturing the incident electron, which is only probable at very
slow incident velocities (low energies).
Radiation Trapping
The direct cross section is a property of an individual atom, and thus should be independent
of how many target atoms are used. However, if the photon emitted by the
excited atom is reabsorbed by another target atom before it reaches the detector,
bad things can happen. This process is called radiation trapping.
Consider the example illustrated in the diagram above. The electron-impact
excited atom-A decays giving off a blue photon. This
blue photon is absorbed by atom-B. Atom-B can then decay giving off either
another blue photon or a green photon. As the pressure (target density)
is increased, reabsorbtion becomes more probable and fewer blue photons can escape
the collision region. At the same time, more and more atoms cascade into the
level-i. Thus, the cascade cross section into level-i is dependent
upon pressure.
back to home page
|